D01: Large-scale dynamical mechanisms of Arctic-midlatitude linkages
PIs: Dörthe Handorf, Christoph Jacobi, Johannes Quaas , (former PI: Markus Rex)
In phase II of (AC)³, atmospheric energy transports into the Arctic and dynamical mechanisms of Arctic midlatitude linkages have been analyzed with respect to the impact of the ocean-atmosphere background state, changing radiative forcing, and representation of stratospheric processes. New machine-learning analysis tools have been developed. We detected preferred pathways of energy transports during synoptic events, and unraveled the impact of changing sea ice concentration (SIC) and sea surface temperatures on Arctic-midlatitude linkages including changes in temperature extremes. We improved the representation of stratospheric waves in the ICOsahedral Non-hydrostatic (ICON) model and their effect of the polar vortex and stratospheric-tropospheric coupling. But there are still large uncertainties about Arctic-midlatitude linkages, with large spreads in climate simulations for Arctic climate, Arctic amplification, and feedback processes. Hence, Arctic amplification processes, and connections of Arctic and midlatitude circulation still have to be improved in global climate models to obtain more reliable climate projections, particularly with respect to Arctic amplification and related Arctic-midlatitude linkages. In phase III, we will study the impact of improved parameterizations, developed in phases I and II of (AC)³, on the simulated Arctic climate and related midlatitude climate linkages in the global ICON model.
Hypothesis:
Advanced representations of Arctic processes in a global climate model improve the representation of Arctic-midlatitude linkages.
Specifically we want to answer the following questions:
- How sensitive is the simulated Arctic climate to changes of surface-related and gravity wave parameterizations?
- How does a better representation of Arctic processes in a global climate model impact the representation of Arctic-midlatitude linkages under present day climate?
- Will a better representation of Arctic processes in a global climate model lead to significant changes in Arctic-midlatitude linkages under future climate conditions?
To answer these questions, we will implement improved physical parameterizations of boundary layer turbulence, surface drag, surface albedo, and gravity wave drag developed previously within (AC)³ into the global ICON model; perform sensitivity simulations for present-day and future climate conditions with the ICON standard version, and with improved parameterizations; evaluate and quantify dynamical processes of Arctic climate change, Arctic amplification and Arctic-midlatitude linkages exploiting reanalyses and (AC)³ observations by applying the methods developed in project D01 during phases I and II. This work plan relates to all of the strategic questions. We will analyze the contributions of new parameterizations to Arctic amplification (SQ1), quantify the effect on Arctic-midlatitude linkages (SQ2) and analyze their role in the future Arctic amplification evolution (SQ3).
Achievements phase II
- Analysis of the role of horizontal energy transports to synoptic events and LRF.
- Unraveling the impact of changing SIC and SSTs on Arctic-midlatitude linkages including extremes.
- Updated GW representation improves stratospheric circulation in ICON.
- New machine learning methods for circulation pattern detection and analysis.
Achievements phase I
D01 has analysed the horizontal transports of moist static energy into the Arctic using an innovative, self-organising maps algorithm (Mewes and Jacobi, 2019). The impact of Arctic sea-ice decline on the large-scale atmospheric circulation has been analysed in reanalysis data and climate model simulations (Romanowsky et al., 2019). A tropospheric pathway mainly occurs in autumn to early winter and results in more frequent occurrence of blockings over Scandinavia and northern Eurasia. This initiates a stratospheric pathway with enhanced upward propagation of energy to weaken the stratospheric polar vortex. The subsequent downward propagation of these stratospheric circulation anomalies in late winter (Jaiser et al., 2016) contributes to persistent negative North Atlantic Oscillation (NAO) anomalies. An improvement of modelled stratospheric pathway for Arctic-mid-latitude linkages by including interactive stratospheric ozone chemistry into general circulation models was achieved (Romanowsky et al., 2019).
Role within (AC)³
Members
Sina Mehrdad
PhD
University of Leipzig
Leipzig Institute for Meteorology (LIM)
Stephanstr. 3
04103 Leipzig
Prof. Dr. Johannes Quaas
Principal Investigator
University of Leipzig
Leipzig Institute for Meteorology (LIM)
Stephanstr. 3
04103 Leipzig
Prof. Dr. Christoph Jacobi
Principal Investigator
University of Leipzig
Leipzig Institute for Meteorology (LIM)
Stephanstr. 3
04103 Leipzig
Ines Höschel
PhD
Alfred Wegener Institute
Telegrafenberg A45
14473 Potsdam
Dr. Dörthe Handorf
Principal Investigator
Alfred Wegener Institute
Telegrafenberg A45
14473 Potsdam
Former Members
Dr. Markus Rex
Principal Investigator
Alfred-Wegener-Institute Helmholtz Center for Polar and Marine Research
Telegrafenberg A45
14473 Potsdam
Dr. Daniel Mewes
PhD (in phase I)
University of Leipzig
Leipzig Institute for Meteorology (LIM)
Stephanstr. 3
04103 Leipzig
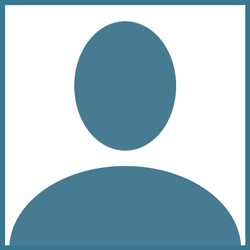
Dr. Daniel Kreyling
PostDoc
Alfred Wegener Institute
Telegrafenberg A45-N
14473 Potsdam
Publications
2024
2023
Galytska, E., Weigel, K., Handorf, D., Jaiser, R., Köhler, R., Runge, J., &; Eyring, V., 2023. Evaluating causal Arctic-midlatitude teleconnections in CMIP6. J. Geophys. Rese. Atmos., 128, e2022JD037978. https://doi.org/10.1029/2022JD037978
Riebold, J., Richling, A., Ulbrich, U., Rust, H., Semmler, T., and Handorf, D., 2023: On the linkage between future Arctic sea ice retreat, Euro-Atlantic circulation regimes and temperature extremes over Europe, Weather Clim. Dynam., 4, 663–682, https://doi.org/10.5194/wcd-4-663-2023.
Karami, K., S. Borchert, R. Eichinger, Ch. Jacobi, A. Kuchar, S. Mehrdad, P. Pisoft, and P. Sacha, 2023: The climatology of elevated stratopause events in the UA-ICON model and the contribution of gravity waves, J. Geophys. Res.: Atmos., 128, e2022JD037907. https://doi.org/10.1029/2022JD037907.
Karami, K.; Garcia, R.; Jacobi, C.; Richter, J. H. & Tilmes, S., 2023: The Holton–Tan mechanism under stratospheric aerosol intervention, Atmos. Chem. Phys., 23, 3799-3818, https://doi.org/10.5194/acp-23-3799-2023
Jaiser, R.; Akperov, M.; Timazhev, A.; Romanowsky, E.; Handorf, D. & Mokhov, I., 2023: Linkages between Arctic and Mid-Latitude Weather and Climate: Unraveling the Impact of Changing Sea Ice and Sea Surface Temperatures during Winter, Meteorol. Z., Schweizerbart Science Publishers, http://doi.org/10.1127/metz/2023/1154
Kirbus, B.; Tiedeck, S.; Camplani, A.; Chylik, J.; Crewell, S.; Dahlke, S.; Ebell, K.; Gorodetskaya, I.; Griesche, H.; Handorf, D.; Höschel, I.; Lauer, M.; Neggers, R.; Rückert, J.; Shupe, M. D.; Spreen, G.; Walbröl, A.; Wendisch, M. & Rinke, A., 2023: Surface impacts and associated mechanisms of a moisture intrusion into the Arctic observed in mid-April 2020 during MOSAiC, Front. Earth Sci., 11, https://doi.org/10.3389/feart.2023.1147848
Wendisch, M.; Brückner, M.; Crewell, S.; Ehrlich, A.; Notholt, J.; Lüpkes, C.; Macke, A.; Burrows, J. P.; Rinke, A.; Quaas, J.; Maturilli, M.; Schemann, V.; Shupe, M. D.; Akansu, E. F.; Barrientos-Velasco, C.; Bärfuss, K.; Blechschmidt, A.-M.; Block, K.; Bougoudis, I.; Bozem, H.; Böckmann, C.; Bracher, A.; Bresson, H.; Bretschneider, L.; Buschmann, M.; Chechin, D. G.; Chylik, J.; Dahlke, S.; Deneke, H.; Dethloff, K.; Donth, T.; Dorn, W.; Dupuy, R.; Ebell, K.; Egerer, U.; Engelmann, R.; Eppers, O.; Gerdes, R.; Gierens, R.; Gorodetskaya, I. V.; Gottschalk, M.; Griesche, H.; Gryanik, V. M.; Handorf, D.; Harm-Altstädter, B.; Hartmann, J.; Hartmann, M.; Heinold, B.; Herber, A.; Herrmann, H.; Heygster, G.; Höschel, I.; Hofmann, Z.; Hölemann, J.; Hünerbein, A.; Jafariserajehlou, S.; Jäkel, E.; Jacobi, C.; Janout, M.; Jansen, F.; Jourdan, O.; Jurányi, Z.; Kalesse-Los, H.; Kanzow, T.; Käthner, R.; Kliesch, L. L.; Klingebiel, M.; Knudsen, E. M.; Kovács, T.; Körtke, W.; Krampe, D.; Kretzschmar, J.; Kreyling, D.; Kulla, B.; Kunkel, D.; Lampert, A.; Lauer, M.; Lelli, L.; von Lerber, A.; Linke, O.; Löhnert, U.; Lonardi, M.; Losa, S. N.; Losch, M.; Maahn, M.; Mech, M.; Mei, L.; Mertes, S.; Metzner, E.; Mewes, D.; Michaelis, J.; Mioche, G.; Moser, M.; Nakoudi, K.; Neggers, R.; Neuber, R.; Nomokonova, T.; Oelker, J.; Papakonstantinou-Presvelou, I.; Pätzold, F.; Pefanis, V.; Pohl, C.; van Pinxteren, M.; Radovan, A.; Rhein, M.; Rex, M.; Richter, A.; Risse, N.; Ritter, C.; Rostosky, P.; Rozanov, V. V.; Donoso, E. R.; Saavedra-Garfias, P.; Salzmann, M.; Schacht, J.; Schäfer, M.; Schneider, J.; Schnierstein, N.; Seifert, P.; Seo, S.; Siebert, H.; Soppa, M. A.; Spreen, G.; Stachlewska, I. S.; Stapf, J.; Stratmann, F.; Tegen, I.; Viceto, C.; Voigt, C.; Vountas, M.; Walbröl, A.; Walter, M.; Wehner, B.; Wex, H.; Willmes, S.; Zanatta, M. & Zeppenfeld, S., 2023: Atmospheric and Surface Processes, and Feedback Mechanisms Determining Arctic Amplification: A Review of First Results and Prospects of the (AC)³ Project, Bull. Am. Meteorol. Soc., American Meteorological Society, 104, E208–E242, https://doi.org/10.1175/bams-d-21-0218.1
Linke, O., Quaas, J., Baumer, F., Becker, S., Chylik, J., Dahlke, S., Ehrlich, A., Handorf, D., Jacobi, C., Kalesse-Los, H., Lelli, L., Mehrdad, S., Neggers, R. A. J., Riebold, J., Saavedra Garfias, P., Schnierstein, N., Shupe, M. D., Smith, C., Spreen, G., Verneuil, B., Vinjamuri, K. S., Vountas, M., and Wendisch, M., 2023: Constraints on simulated past Arctic amplification and lapse rate feedback from observations, Atmos. Chem. Phys., 23, 9963–9992, https://doi.org/10.5194/acp-23-9963-2023.
2022
Karami K., S. Mehrdad, and Ch. Jacobi, 2022: Response of the resolved planetary wave activity and amplitude to turned off gravity waves in the UA-ICON general circulation model, J. Atmos. Sol.-Terr. Phys., 241, 105967, https://doi.org/10.1016/j.jastp.2022.105967.
Schneider, T., C. Lüpkes, W. Dorn, D. Chechin, D. Handorf, S. Khosravi, V.M. Gryanik, I. Makhotina, and A. Rinke, 2022: Sensitivity to changes in the surface-layer turbulence parameterization for stable conditions in winter: A case study with a regional model over the Arctic, Atm. Sci. Lett., 23, e1066, https://doi.org/10.1002/asl.1066
2021
A. Rinke, J. J. Cassano, E. N. Cassano, R. Jaiser, D. Handorf, 2021; Meteorological conditions during the MOSAiC expedition: Normal or anomalous?. Elementa-Sci. Anthrop. 9 (1): 00023. doi: https://doi.org/10.1525/elementa.2021.00023
Kretzschmar, J., 2021: Improving the representation of Arctic clouds in atmospheric models across scales using observations, Dissertation, Universität Leipzig, https://nbn-resolving.org/urn:nbn:de:bsz:15-qucosa2-752400.
Köhler, R., D. Handorf, Ralf Jaiser, Klaus Dethloff, Günther Zängl, Detlev Majewski, Markus Rex, 2021: Improved Circulation in the Northern Hemisphere by Adjusting Gravity Wave Drag Parameterizations in Seasonal Experiments With ICON-NWP, Earth and Space Sci., 8, e2021EA001676, https://doi.org/10.1029/2021EA001676.
Mewes, D., 2021: Large-scale Horizontal Energy Fluxes into the Arctic Analyzed Using Self-organizing Maps, Dissertation, Universität Leipzig, https://nbn-resolving.org/urn:nbn:de:bsz:15-qucosa2-751794.
Wendisch, M., D. Handorf, I. Tegen, R. A. J. Neggers, and G. Spreen, 2021, Glimpsing the ins and outs of the Arctic atmospheric cauldron, Eos, 102, https://doi.org/10.1029/2021EO155959. Published on 16 March 2021.
Köhler, R., Handorf, D., Jaiser, R., Dethloff, K., Zängl, G., Majewski, D., & Rex, M., 2021. Improved circulation in the Northern Hemisphere by adjusting gravity wave drag parameterizations in seasonal experiments with ICON-NWP. Earth Space Sci., 8, e2021EA001676. https://doi.org/10.1029/2021EA001676
2020
D. Mewes and C. Jacobi, 2020: Horizontal Temperature Fluxes in the Arctic in CMIP5 Model Results Analyzed with Self-Organizing Maps, Atmosphere, vol. 11, no. 3, doi: 10.3390/atmos11030251.
J. Cohen, X. Zhang, J. Francis, T. Jung, R. Kwok, J. Overland, T. Ballinger, U.S. Bhatt, H. W. Chen, D. Coumou, S. Feldstein, D. Handorf, G. Henderson, M. Ionita, M. Kretschmer, F. Laliberte, S. Lee, H. W. Linderholm, W. Maslowski, Y. Peings, K. Pfeiffer, I. Rigor, T. Semmler, J. Stroeve, P.C. Taylor, S. Vavrus, T. Vihma, S. Wang, M. Wendisch, Y. Wu, J. Yoon, 2020: Divergent consensuses on Arctic amplification influence on midlatitude severe winter weather. Nat. Clim. Chang. 10, 20–29, doi:10.1038/s41558-019-0662-y. https://www.nature.com/articles/s41558-019-0662-y
2019
Dethloff, K., Handorf, D., Jaiser, R. and Rinke, A., 2019, Kältere Winter durch abnehmendes arktisches Meereis. Phys. Unserer Zeit, 50: 290-297. doi:10.1002/piuz.201901547
Vihma, T., R. Graversen, L. Chen, D. Handorf, N. Skific, J.A. Francis, N. Tyrrell, R. Hall, E. Hanna, P. Uotila, K. Dethloff, A.Y. Karpechko, H. Björnsson, J.E. Overland, 2019: Effects of the tropospheric large‐scale circulation on European winter temperatures during the period of amplified Arctic warming, accepted for publication in International Journal of Climatology, doi:10.1002/joc.6225
Rinke, A., E. Knudsen, D. Mewes, W. Dorn, D. Handorf, K. Dethloff, J.C. Moore, 2019: Arctic summer sea-ice melt and related atmospheric conditions in coupled regional climate model simulations, J. Geophys. Res., 124, doi:10.1029/2018JD030207
Romanowsky, E., D. Handorf, M. Rex, R. Jaiser, I. Wohltmann, W. Dorn, J. Ukita, J. Cohen, and K. Dethloff, 2019: The role of stratospheric ozone for Arctic-midlatitude linkages, Nature Scientific Reports, 9, Article 7962, doi:10.1038/s41598-019-43823-1
Mewes, D., and C. Jacobi, 2019: Heat Transport Pathways into the Arctic and their Connections to Surface Air Temperatures, Atmos. Chem. Phys., 19, 3927-3937, doi:10.5194/acp-19-3927-2019
Dethloff, K., D. Handorf, R. Jaiser, A. Rinke, P. Klinghammer, 2019: Dynamical mechanisms of Arctic amplification, Annals of New York Academy of Sciences, 1436, doi:10.1111/nyas.13698
2018
Kreyling, D., Wohltmann, I., Lehmann, R., and Rex, M., 2018: The Extrapolar SWIFT model (version 1.0): Fast stratospheric ozone chemistry for global climate models, Geosci. Model Dev., 11, 753-769, doi:10.5194/gmd-11-753-2018
Jacobi, Ch., T. Ermakova, D. Mewes, and A.I. Pogoreltsev, 2017: El Niño influence on the mesosphere/lower thermosphere circulation at midlatitudes as seen by a VHF meteor radar at Collm (51.3°N, 13°E), Adv. Radio Sci., 15, 199-206, doi:10.5194/ars-15-199-2017
Stober, G., Matthias V. , Jacobi Ch., Wilhelm S., Höffner J., Chau J.L., 2017: Exceptionally strong summer-like zonal wind reversal in the upper mesosphere during winter 2015/16, Ann. Geophys., 35, 711-720, doi:10.5194/angeo-35-711-201
Wendisch, M., M. Brückner, J. P. Burrows, S. Crewell, K. Dethloff, K. Ebell, Ch. Lüpkes, A. Macke, J. Notholt, J. Quaas, A. Rinke, and I. Tegen, 2017: Understanding causes and effects of rapid warming in the Arctic. Eos, 98, doi:10.1029/2017EO064803